Comprehensive Analysis of Blasting Technology: From Principles and Classification to Industrial Applications
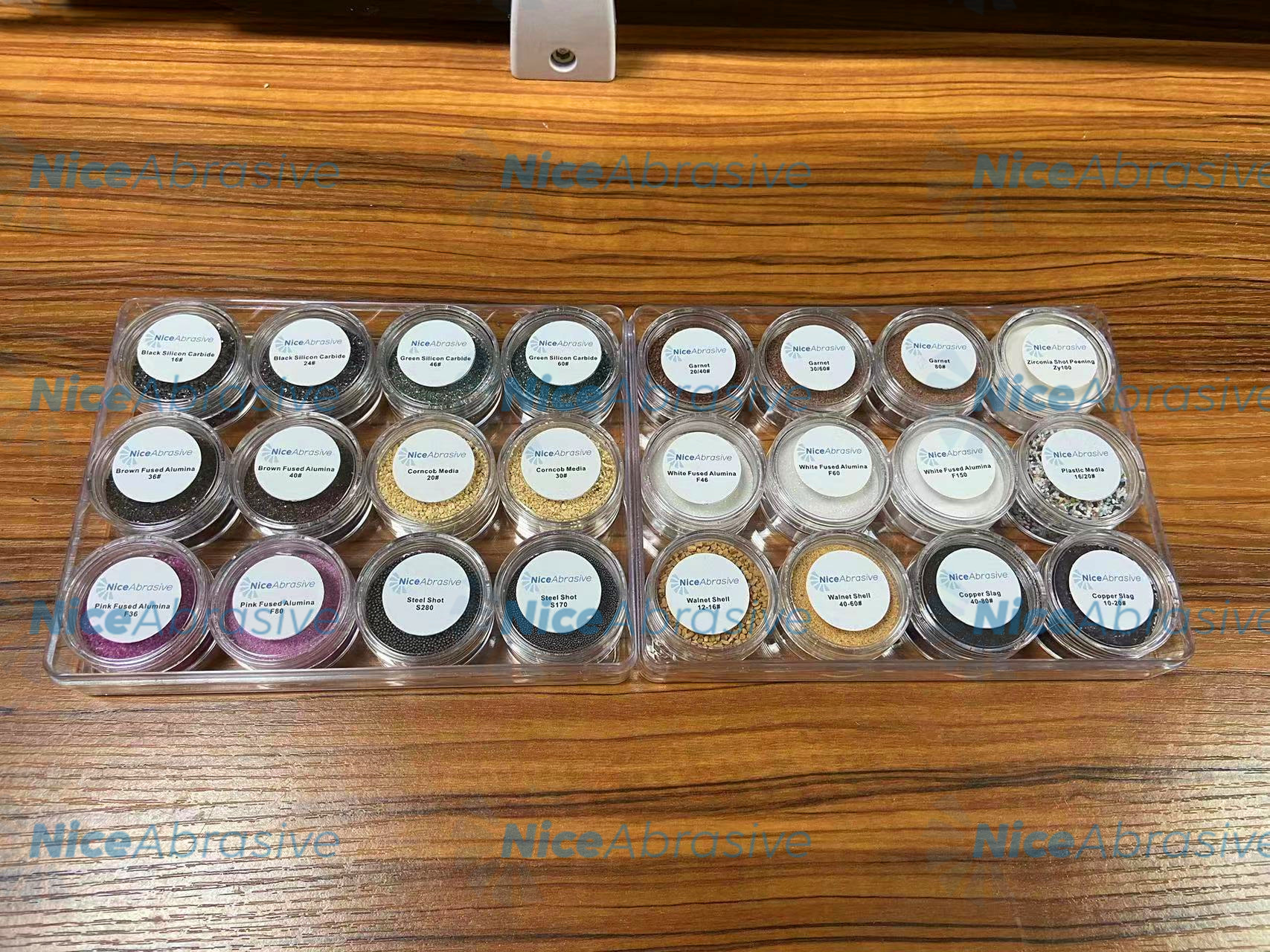
1. Origins and Core Essence of Blasting Technology
Blasting, a time-honored surface treatment technique, traces its origins to the 1870s. Inspired by a coastal sandstorm that frosted glass surfaces, American engineer B.C. Tillman invented the first “sandblasting device” using emery as an abrasive, laying the foundation for this physical processing method. Over a century, blasting has evolved from manual operation to a precision manufacturing system integrating aerodynamics, materials science, and automation, widely applied to surface treatment of metals, glass, ceramics, plastics, and other materials.
At its core, blasting belongs to the category of “free-abrasive machining,” distinguished from “fixed-abrasive machining” (e.g., belt grinding, sandpaper polishing) by using free-state (dry or wet) abrasives propelled at high velocity to impact workpieces. This physical impact, scraping, or friction modifies surfaces without relying on chemical reagents or fixed abrasives, offering flexibility and efficiency that make it one of industry’s most versatile surface treatment methods.
2. Classification and Technical Characteristics of Blasting Processes
2.1 Dry Blasting vs. Wet Blasting: Core Environmental Dimensions
Based on abrasive environment, blasting is broadly categorized into two systems:
(1) Dry Blasting
- Typical Methods: Sandblasting, blower blasting, suction blasting, dry ice blasting, shot blasting.
- Technical Features:
- Energy Source: Pneumatic (compressor-driven) or mechanical (centrifugal impeller-driven).
- Applications: Suitable for moisture-sensitive workpieces (e.g., electronics) or high-impact scenarios (e.g., heavy machinery rust removal).
- Limitations: Severe dust pollution, abrasive residue risks, requiring dust collection systems.
(2) Wet Blasting
- Typical Methods: Liquid honing, wet shot blasting.
- Technical Features:
- Medium: Abrasives mixed with water to form a slurry, propelled by compressed air or centrifugal force.
- Key Advantages: Reduces dust emissions by >90%, ensures uniform abrasive distribution, and handles micron-scale precision tasks; simultaneously cleans and processes surfaces, ideal for heat-sensitive or high-precision materials (e.g., aerospace aluminum alloys).
- Limitations: Unsuitable for water-sensitive workpieces; equipment requires anti-corrosion maintenance.
2.2 Power Types: Differences Between Air-Driven and Mechanical-Driven Systems
Classification | Representative Methods | Energy Source | Velocity Range | Applications | Typical Abrasives |
---|---|---|---|---|---|
Air-Driven (Dry/Wet) | Sandblasting, wet blasting | Compressed air (0.1–0.8MPa) | 20–100 m/s | Localized treatment of complex geometries, precision cleaning | Aluminum oxide, glass beads, walnut shell |
Mechanical-Driven (Dry/Wet) | Shot blasting, wet shot blasting | Centrifugal impeller (2000–6000 RPM) | 50–150 m/s | High-throughput treatment of large surfaces, heavy-duty deburring | Steel shot, stainless steel shot |
2.3 Specialized Blasting Technologies: Innovative Applications
- Dry Ice Blasting: Uses solid CO₂ particles that sublimate upon impact, leaving no residue. Ideal for cleaning food processing equipment and precision electronics.
- Suction Blasting: Employs negative pressure to adsorb abrasives in sealed environments, achieving zero dust emission—critical for enclosed spaces like pipeline interiors.
- Wet Shot Blasting: Combines wet blasting’s cleaning advantages with shot blasting’s efficiency, currently in development for applications like deburring new energy vehicle battery casings.
3. Core Application Scenarios of Blasting Technology
3.1 Surface Pretreatment: Preparing for Subsequent Processes
- Rust and Scale Removal:
Blasting efficiently removes rust (Fe₂O₃·nH₂O) and mill scale (e.g., Fe₃O₄ on steel) from metal workpieces. For example, shot blasting ship steel plates before painting increases coating adhesion by 3–5 times. - Paint and Coating Stripping:
Unlike chemical strippers, blasting removes old coatings from metals and concrete without residue, making it ideal for heritage restoration or eco-friendly scenarios (e.g., automotive recycling).
3.2 Surface Functional Modification: Imparting New Properties
- Roughing and Anchor Effect:
Blasting creates micron-scale surface roughness (anchor pattern depth 20–100μm) to enhance bonding strength in welding or adhesive applications. For instance, glass bead blasting aluminum automotive components increases adhesive contact area by 40%. - Shot Peening:
High-velocity impact from spherical abrasives (e.g., steel/ceramic beads) induces compressive residual stresses (50–500μm depth), boosting fatigue life by 5–10 times. Widely used in critical load-bearing components like aircraft turbine blades and automotive springs.
3.3 Precision Machining and Artistic Creation
- Deburring and Edge Rounding:
Fine-grit abrasives (e.g., 200-mesh aluminum oxide) remove micro-burrs (<0.1mm) from precision gears and medical devices, ensuring consistency unmatched by manual grinding. - Surface Texturing:
Blasting enables diverse finishes on glass and stone—from matte to Mirror (mirror-like)—by controlling abrasive grit and spray angle. For example, 100-mesh silicon carbide creates uniform frosted glass, while 500-mesh cerium oxide achieves localized polishing.
4. Advantages and Challenges of Blasting Technology
4.1 Core Advantages: The Unique Value of Physical Processing
- Material Universality:
Applicable to metals, non-metals, conductors, and insulators, including surface activation of carbon fiber composites. - Non-Directional Uniformity:
Random abrasive impacts create isotropic surface textures, avoiding the directional patterns of traditional machining (e.g., milling). This is critical for optics and aerospace components requiring specific light reflection or aerodynamic properties. - Process Flexibility:
Single equipment can switch between heavy-duty rust removal and fine polishing by changing abrasives, pressure, or nozzle angles, reducing capital investment for manufacturers.
4.2 Challenges: Directions for Technical Optimization
- Geometric Limitations:
Deep holes (<1mm diameter) and complex internal cavities are hard to reach, requiring robotic guidance or vibration-assisted blasting. - Equipment Wear and Maintenance:
High-hardness abrasives (e.g., silicon carbide) wear nozzles and impellers rapidly; tungsten carbide nozzles may need replacement every 50 hours, increasing downtime costs. - Environmental Impact:
Dry blasting emits respirable silica dust (a health hazard), necessitating HEPA filtration; wet blasting wastewater requires sedimentation and filtration to meet discharge standards.
5. Scientific Logic of Abrasive Selection
5.1 Three Key Abrasive Factors: Material, Grit Size, and Shape
Dimension | Selection Criteria | Case Studies |
---|---|---|
Material | Abrasive hardness must match workpiece: soft materials (e.g., plastic) use glass beads; hard materials (e.g., alloy steel) use silicon carbide. | Stainless steel shot for aluminum wheels prevents iron contamination. |
Grit Size | Coarse grit (<40 mesh) for heavy rust removal; fine grit (>200 mesh) for precision polishing. | 500-mesh zirconia for medical device polishing. |
Shape | Angular abrasives (e.g., brown fused alumina) for aggressive deburring; spherical abrasives (e.g., steel shot) for peening. | Φ0.8mm steel shot for automotive gear peening to form uniform compressive stress layers. |
5.2 Environmental Trends: Rise of Sustainable Abrasives
- Biodegradable Abrasives: Corn cob and walnut shell abrasives are ideal for food-grade equipment cleaning, eliminating chemical residue risks.
- Recycling Technologies: Magnetic separation and screening recover used steel shot, which is re-tempered for reuse, reducing abrasive costs by 30–50%.
6. Future Technological Evolution: Intelligence and Greenization
6.1 Intelligent Blasting Systems
Integrated sensors and AI algorithms monitor real-time abrasive flow, pressure, and surface roughness to auto-adjust parameters. For example, a Swiss-developed robotic blasting cell uses vision systems to dynamically plan paths for complex curves, improving efficiency by 40%.
6.2 Low-Energy Technologies
Blower blasting replaces compressors with centrifugal fans, cutting energy consumption by 60%, suitable for low-intensity surface texturing (e.g., wood).
6.3 Advanced Dry Dust Collection
Pulse-jet bag filters combined with cyclone separators capture 99.9% of particles ≥2.5μm, meeting ISO 14001 standards for dust-intensive industries like aluminum profile manufacturing.
6.4 Nanoblasting and Microscale Applications
Emerging nanoblasting techniques use submicron abrasives (e.g., diamond nanoparticles) for precision surface modification in microelectronics, such as roughening semiconductor packaging materials without damaging delicate structures.
7. Case Studies: Industrial Applications Across Sectors
7.1 Aerospace Industry
- Composite Component Processing:
Wet blasting with 120-mesh glass beads removes oxidation layers from carbon fiber composite aircraft panels, enhancing adhesive bonding for honeycomb core assembly. - Turbine Blade Refurbishment:
Dry ice blasting cleans heat-resistant coatings from turbine blades without damaging superalloy substrates, reducing maintenance time by 50% compared to chemical cleaning.
7.2 Automotive Manufacturing
- EV Battery Housing Production:
Robotic wet blasting systems with 200-mesh plastic media deburr aluminum battery casings, ensuring zero metal debris while achieving Ra 1.6μm surface finish. - Powertrain Components:
High-pressure dry blasting (0.6MPa) with steel grit removes casting flash from engine blocks, preparing surfaces for non-destructive testing (NDT) with 99% defect detection accuracy.
7.3 Medical Device Manufacturing
- Orthopedic Implant Surface Engineering:
Plasma-nitrided titanium hip stems undergo ultrasonic wet blasting with 500-mesh hydroxyapatite particles, creating nano-roughness (Ra 0.8–1.2μm) to promote osseointegration. - Surgical Tool Deburring:
Micro-blasting (0.2mm nozzle) with 1000-mesh alumina powder removes burrs from laparoscopic instrument hinges, ensuring smooth operation with <5μm tolerance.
8. Cost-Benefit Analysis and Process Optimization
8.1 Cost Structure Breakdown
Cost Category | Dry Blasting | Wet Blasting |
---|---|---|
Abrasives | 25–40% of total cost (high consumption) | 15–25% (recyclable slurry systems) |
Equipment Depreciation | 30–40% (due to wear parts) | 35–45% (requires corrosion-resistant components) |
Labor & Energy | 15–20% (high compressor usage) | 20–25% (water recycling systems) |
Environmental Compliance | 10–15% (dust collection) | 15–20% (wastewater treatment) |
8.2 ROI Case: Automotive Component Manufacturer
A Tier 1 supplier adopted automated wet blasting for engine crankshafts:
- Initial Investment: $250,000 for a robotic system.
- Annual Savings:
- 60% reduction in post-blasting cleaning labor.
- 80% decrease in abrasive waste (slurry recycling).
- 95% improvement in surface defect detection prior to painting.
- Payback Period: 18 months, with long-term savings from reduced warranty claims due to improved coating adhesion.
9. Technical Challenges and Research Frontiers
9.1 Tribomechanical Modeling
Advanced finite element analysis (FEA) models simulate abrasive impact dynamics, predicting surface roughness and stress distribution with <5% error. Researchers at MIT have developed multiscale models combining molecular dynamics (MD) and continuum mechanics to optimize abrasive trajectories.
9.2 Hybrid Processing Systems
Combining blasting with other surface treatments:
- Blasting + Laser Texturing: Sequential processing creates hierarchical surface structures for enhanced tribological performance (e.g., reduced friction in engine cylinders).
- Blasting + Electropolishing: Post-blasting electrochemical polishing achieves mirror finishes on medical implants while retaining beneficial micro-roughness for cell adhesion.
9.3 Sustainable Abrasive Development
- Bio-Based Abrasives: Researchers at ETH Zurich have developed biodegradable abrasives from waste oyster shells, with hardness comparable to glass beads and zero environmental impact.
- Magnetic Abrasives: Iron-based particles mixed with magnetic fluids enable controlled blasting in complex geometries using external magnetic fields, minimizing over-treatment.
10. Conclusion: Strategic Positioning of Blasting Technology
Blasting is not a one-size-fits-all solution, but its physical processing advantages make it indispensable for surface pretreatment, functional modification, and precision manufacturing. Key considerations for industrial adoption include:
- Workpiece Complexity: Air-driven wet blasting for intricate geometries; mechanical-driven dry blasting for large, simple parts.
- Performance Requirements: Spherical abrasives for peening; angular abrasives for aggressive cleaning.
- Sustainability Goals: Wet systems for low-dust environments; recycled abrasives for circular economy compliance.
As materials science and Industry 4.0 advance, blasting will evolve toward smarter, greener, and more precise applications. For customized solutions, collaboration with technology providers to conduct process trials and validate parameters remains critical. By embracing innovation, manufacturers can leverage blasting as a strategic enabler for high-quality, sustainable production.
References
[1] Tillman, B.C. (1870). Method of and apparatus for polishing, matting, and etching glass and other surfaces. U.S. Patent 108,408.
[2] ASM International. (2019). Handbook of Surface Engineering. Materials Park, OH: ASM International.
[3] ISO 11126-2:2017. Preparation of steel substrates before application of paints and related products — Specifications for abrasives for blasting — Part 2: Ferrous abrasives.
[4] Wu, S. et al. (2022). A review of intelligent blasting technologies in manufacturing. Journal of Manufacturing Systems, 64, 550–567.
[5] Environmental Protection Agency (EPA). (2020). Control of Respirable Crystalline Silica in Blasting Operations. OSHA Standard 29 CFR 1910.1053.
[1] Tillman, B.C. (1870). Method of and apparatus for polishing, matting, and etching glass and other surfaces. U.S. Patent 108,408.
[2] ASM International. (2019). Handbook of Surface Engineering. Materials Park, OH: ASM International.
[3] ISO 11126-2:2017. Preparation of steel substrates before application of paints and related products — Specifications for abrasives for blasting — Part 2: Ferrous abrasives.
[4] Wu, S. et al. (2022). A review of intelligent blasting technologies in manufacturing. Journal of Manufacturing Systems, 64, 550–567.
[5] Environmental Protection Agency (EPA). (2020). Control of Respirable Crystalline Silica in Blasting Operations. OSHA Standard 29 CFR 1910.1053.